The ADS GC-MS system was extremely successful as the first automated GC-MS instrument to be deployed long-term at remote stations. Because it depends on Peltier thermoelectric devices for cooling, trapping of analytes with the ADS system can not be done below a temperature of -50˚C. At this temperature there are unavoidable trade-offs in the design of the trapping system. Increasing trap size and the amounts of absorbents reduces the bleed-through of the most volatile analytes and increases the size of air samples that can be analyzed. However, with large amount of absorbents in the trap it also becomes increasingly difficult to maintain sharp and reproducible injections into the GC-MS over the wide range of volatilities of the compounds being measured. The current ADS preconcentration system represents an optimal solution within these constraints, but because of its limited trapping temperature, we have been unable to find absorbents that would permit the system to retain quantitatively the most volatile perfluorinated compounds that are of importance climatically and in the context of the Kyoto Protocol.
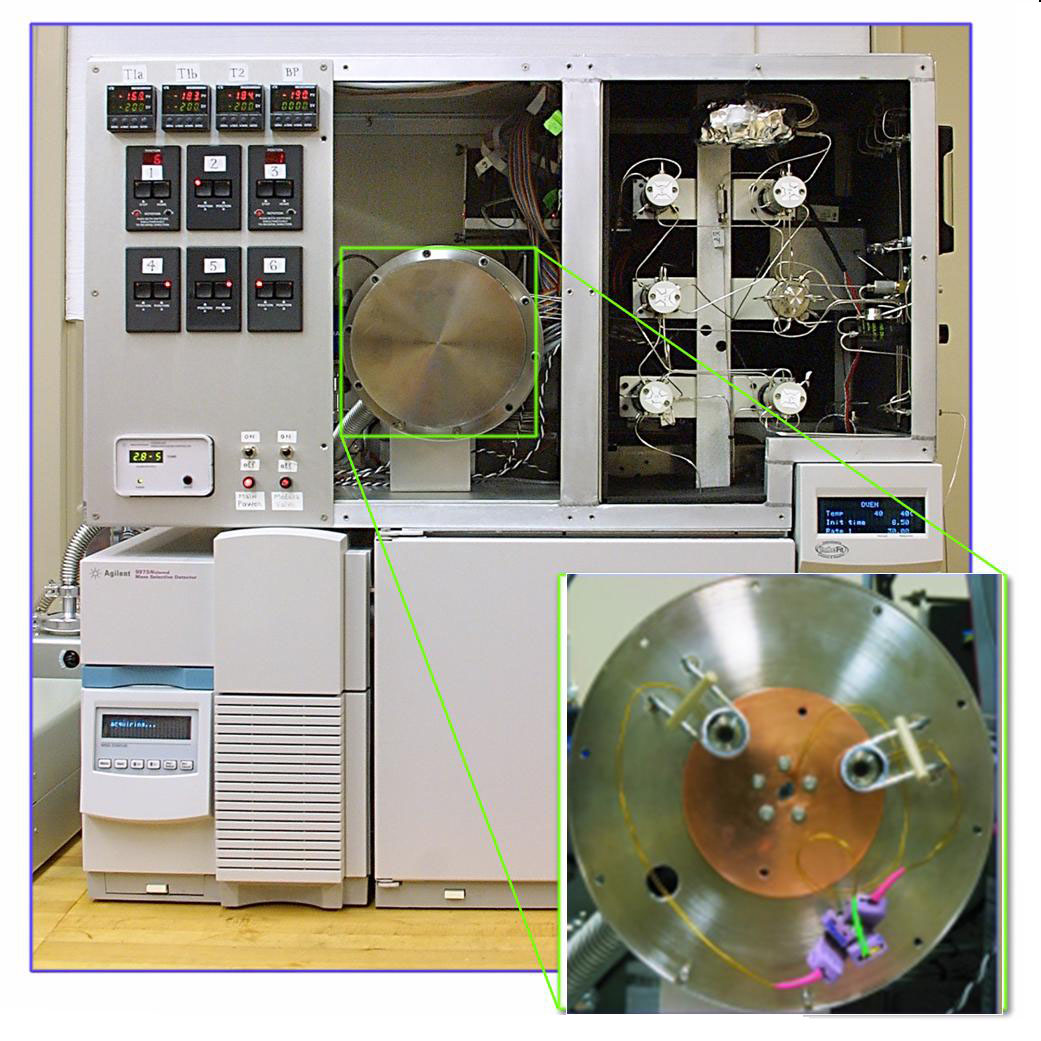
Figure 1. Front view of the Medusa sample module mounted on an Agilent 5973 GC-MS, with the two front service panels removed. Temperature and Valco valve controllers are mounted on the left. The opening on the right shows the thermostatted enclosure housing the Valco valves and other flow components. The opening in the center shows the cryogenic vacuum chamber. Inset: interior of cryogenic vacuum chamber with the two traps mounted on the copper cold plate.
In order to address these concerns, the SIO AGAGE laboratory, with strong input from the University of Bristol group, developed a new GC-MS cryogenic preconcentration system called Medusa (Figure 1) after the appearance of its early prototypes (see Miller et al., 2008 for details). At the heart of the Medusa is a Polycold "Cryotiger" cold end which maintains a temperature of -175˚C, even with a substantial heat load, using a simple single-stage compressor with a proprietary mixed-gas refrigerant. This cold end conductively cools two traps to about -165˚C. By using standoffs of limited thermal conductivity to connect the traps to the cold head, each trap can be independently heated resistively to any temperature from -165˚C to +200˚C or more, while the cold end remains cold. The use of two traps with wide programmable temperatures ranges, coupled with the development of appropriate trap absorbents, permits the desired analytes from 2-liter air samples to be effectively separated from more-abundant gases that would otherwise interfere with chromatographic separation or mass spectrometric detection, such as N2, O2, Ar, H2O, CO2, CH4, Kr and Xe. Importantly, the dual traps also permit the analytes to be purified of interfering compounds by fractional distillation and re-focusing from the larger first-stage trap (T1) onto a smaller trap (T2) (Figure 2) at very low temperatures, so that the resulting injections to the Agilent 5973 GC-MS are sharp and reproducible. By trapping and eluting analytes at very low temperatures, the range of compounds that can be measured is greatly extended to include a number of important volatile compounds, and problems with reaction of analytes on the traps at higher temperatures are avoided. The Medusa system uses a high-precision integrating mass flow controller (MFC) for improved measurement of sample volumes. In addition, significant advances have been made in the software to control and acquire data from the Medusa and the GC-MS itself, so that the entire system has programmability, versatility and ease of operation comparable to that of the AGAGE GC-MD instruments.
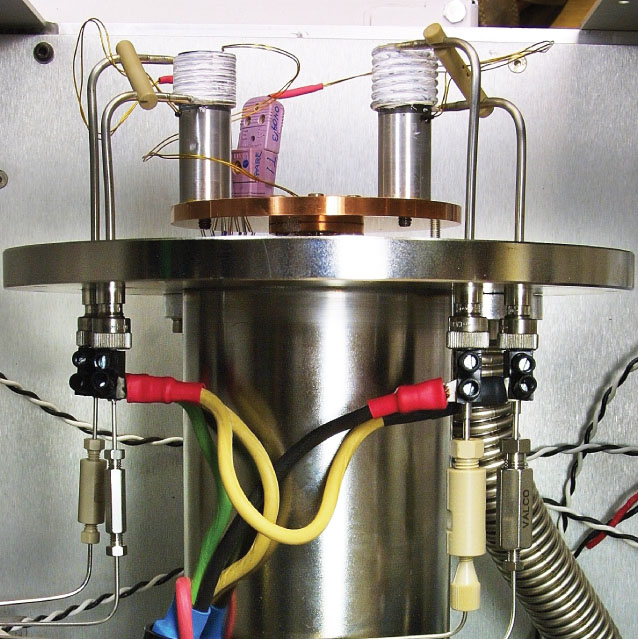
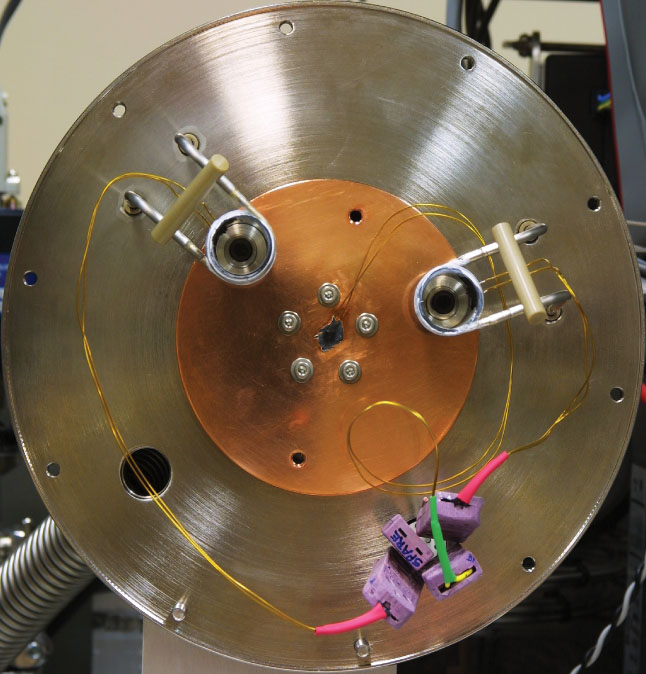
Figure 2. Top view (a) and front view (b) of the Medusa cryogenic vacuum chamber with the cover removed. The two traps T1 and T2 mounted to the continuously cooled copper baseplate using thin aluminum thimbles which permit the two traps to be heated independently while the baseplate remains cold.
As with the ADS, the Medusa measures 2-liter air samples, but at a frequency (60 minutes per measurement) that is twice that of the ADS. A list of the 40 trace gas species it currently measures, ranging in concentration from a few tenths of a ppt (part-per-trillion) to about 600 ppt, is given in Table 1, together with typical measurement precisions. The two traps, T1 and T2 (Figures 2a and 2b) are packed with HayeSep-D, a relatively inert high-surface-area polymer, but the less-volatile analytes never contact this adsorbent because they are trapped in the wraps of -165˚C open tubing before they reach the absorbent and are subsequently transferred by backflushing.
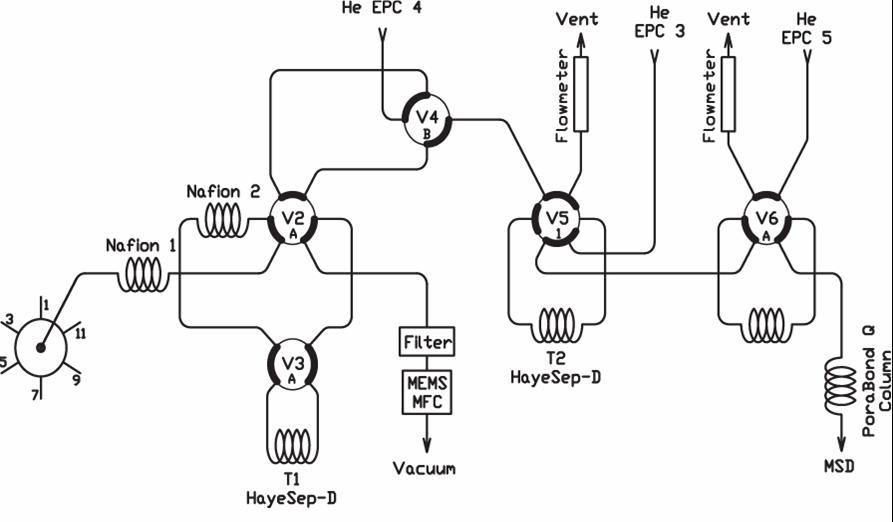
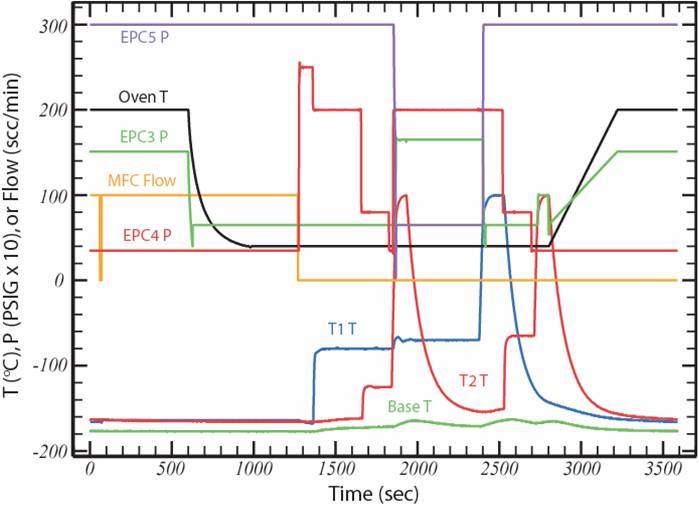
Figure 3. The flow scheme of the Medusa (a) allows samples from the 6 inlets on the left to be dried, trapped and fractionally distilled using the two traps, and then injected for GC-MS analysis. EPCs are electronic pressure controllers, the Vs are multiport valves, the MFC is a mass flow controller, and the MSD is a mass-selective detector. The "strip chart" (b) shows how pressures, temperatures and sample flow are controlled during the trapping and analysis.
The Medusa permits several stages of analyte purification and refocusing prior to GC-MS analysis. A sample from any of 6 pressurized inlets flows through two counterpurged Nafion driers before passing through T1 to a filter and MFC (set to 100 scc/min) where the sample volume is determined before exhausting to vacuum. After trapping, T1 is postflushed with helium from electronic pressure controller EPC-4 to further remove N2, O2, Ar and CH4. T1 is then warmed to ~-65˚C and CF4 and NF3 are transferred to T2 using helium. T2 is then postflushed at about -125˚C to further reduce bulk and noble gases. Then T2 is heated to +100˚C and CF4 and NF3 are injected into the GC-MS. While CF4 and NF3 are analyzed, T1 is further postflushed at about -70˚C to reduce CO2 and Xe. T1 is then heated to +100˚C and backflushed with helium to transfer the remaining analytes to T2, which is then postflushed at -65˚C to further reduce CO2 and Xe. Finally, T2 is again heated to +100˚C and the remaining analytes are backflushed into the GC-MS for separation on a PoraBOND Q capillary column, which is ramped from 40˚C to >200˚C during the main analysis to allow less-volatile analytes to elute sharply.
Prior to the discovery of atmospheric NF3 (Weiss et al., 2008), CF4 was the most analytically challenging gas for the Medusa to measure due to its volatility and the difficulty in separating it from the more abundant volatile gases (O2, N2, Ar, Kr, CO2, and CH4). The challenge of measuring CF4 was overcome by Miller et al. (2008) by injection from T2 onto a MS-4A and HiSiv-3000 packed micro-precolumn, which separates CF4 from residual O2, N2, Ar, Kr, and CO2. This methodology, however, was not applicable to NF3 because of its low abundance and poor sensitivity. Arnold et al. (2012) developed a new flow scheme by which CO2 is largely removed during the first T1 to T2 transfer by a MS-4A packed micro-precolumn, allowing direct injection from T2 onto a GasPro capillary column for separation of CF4 and NF3 from the remaining bulk gases, noble gases, CH4 and CO2. The Miller et al. (2008) and Arnold et al. (2012) methods are identical for analysis of all other analytes.
In routine operation of the Medusa systems, there are substantial investments in optimization and data collection and processing. The Agilent GC-MS control, tuning and acquisition software was not up to this task, and has been completely replaced by custom software under the Linux operating system which runs both the Medusa "front end" and the GC-MS in selective ion mode (SIM). This software builds upon the base "gccontrol" developed earlier for the GC-MD, but now includes the mass/charge ratio as a variable, as well as the many control and diagnostic parameters of the Medusa. Blanks and instrument linearities are measured routinely. This software is continually being updated in response to the needs of the station operators and the data processors. As in the case of the GC-MD, the Medusa instruments can now be run from anywhere in the world, and the data are available to all AGAGE investigators.
A number of significant technological hurdles had to be overcome to make the Medusas operational. The design of traps T1 and T2 to have good thermal connection to the cold end baseplate while retaining the electrical isolation necessary for low-voltage high-current resistive heating was overcome by anodizing the Al thimbles on which they are wrapped, and by using thermally conductive silicone paste. The Cryotiger cold ends have an inherent problem with oil vapor blockage which has been greatly ameliorated, but not eliminated, by working closely with the manufacturer to install oil traps in the refrigerant lines. Because of the importance of measuring CF4 and NF3, a great deal of effort has gone into removing CO2 interferences, firstly for CF4 (Miller et al., 2008), and after identification of a growing NF3 abundance (Weiss et al., 2008), for both CF4 and NF3 (Arnold et al., 2012). Because of the high rate of Medusa standard gas consumption (24 liters/day), a quaternary level of whole-air calibration gas was added to the normal tertiary level of calibration used by the GC-MD instruments. The quaternary working gases are calibrated over the course of their use in the field by analyses against the tertiary standards sent from SIO for both the Medusas and GC-MDs at each station. Otherwise, the calibration hierarchy is the same as is used for the GC-MDs (Prinn, Weiss, et al., 2000), including the practice of alternating ambient air and calibration gas analyses to obtain the highest precision measurements.
An important advance in the Medusa is its ability to check its linearity by injecting a wide range of standard gas volumes. Such measurements were used throughout the development process to optimize trapping materials and operating parameters, and they are now part of routine diagnostics which confirm that the Medusas are linear over wide ranges of sample volume, concentration, and composition. Such linearity and composition-independence are critical to accurate calibration, especially when propagating synthetic primary standards or when measuring samples spanning wide concentration ranges.
The Medusa systems are producing exceptional routine precisions to rival those of the other GC-MD systems. By using quantifier (target) and qualifier ions for each measured species, the Medusa also offers improved peak identification and reduced susceptibility to interference by co-eluting species. This is especially important in the case of methyl chloroform, which should be measurable down to ~1 ppt with a precision of ~2%, and thus remain valuable in determining global OH for roughly another two decades.
The original ADS GCMS instruments at Mace Head and Cape Grim were retired in December 2004. The new generation of Medusa GCMS began routine operation in November 2003 at Mace Head and January 2004 at Cape Grim. Three additional Medusa GCMS instruments were also installed and started routine measurements at Trinidad Head, California and Barbados in May 2005, and at American Samoa in May 2006, respectively. Upgrades to Medusa systems to incorporate measurements of NF3 (see Arnold et al., 2012) began in 2013 at Mace Head and Gosan, and in 2014 at Jungfraujoch. Further updates await scheduling.